
We live times of unprecedented vulnerability to natural hazards, which is exacerbated more than ever by extreme climate, population growth, social disparity, and uncontrolled urbanization. In an era of widespread connectivity, we observe in real-time the catastrophic effects of natural disasters, often crystallized by the scenes of devastation reported after earthquakes, floods, landslides or cyclones. Engineering science and technology is set to play a key role in assessing, preventing, and managing natural risks in highly populated urban areas. This thrust focuses on the development of predictive tools to understand how natural settings and urban systems interact. Most importantly, it focuses on the formulation, development and implementation of city-scale models allowing us to track in real-time how natural hazards develop, thereby assisting the design of technologies and the implementation of policies to reduce losses and protect the quality of life.
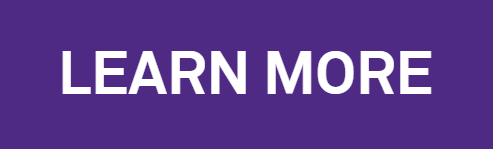
Geomechanics of sand liquefaction
Sands exhibit a wide range of mechanical properties, spanning from those of relatively stiff solids under dry, dense conditions, to those of rapidly moving viscous fluids in the presence of loosely packed, fluid-saturated states. Research conducted at the SPREE center focuses on developing cutting-edge simulation tools bridging the gap between these radically different conditions. The computer models resulting from these activities enable the identification of the natural events able to turn sand deposits into fluids through mechanical instabilities, as well as the quantification of the volume, velocity and travel distance of the mobilized soil mass.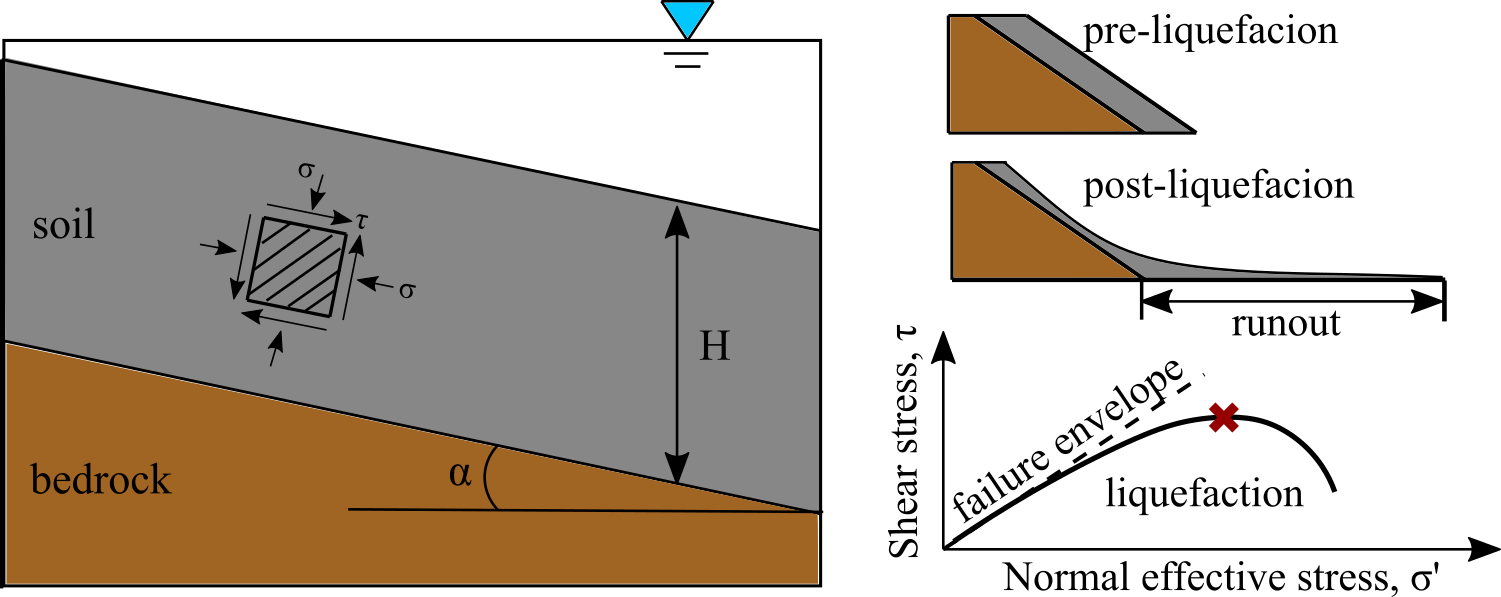
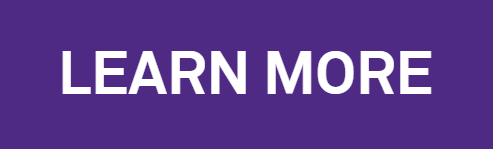
Mechanics-based landslide hazard mapping at regional scale
Management of infrastructural assets and protection of communities inhabiting densely populated urban settings requires the assessment of the intensity, frequency, and magnitude of hazardous events across large areas. Research done at the SPREE center formulates science-based tools to map spatially-varying hazards at the city scale by taking into account natural heterogeneity and system uncertainty.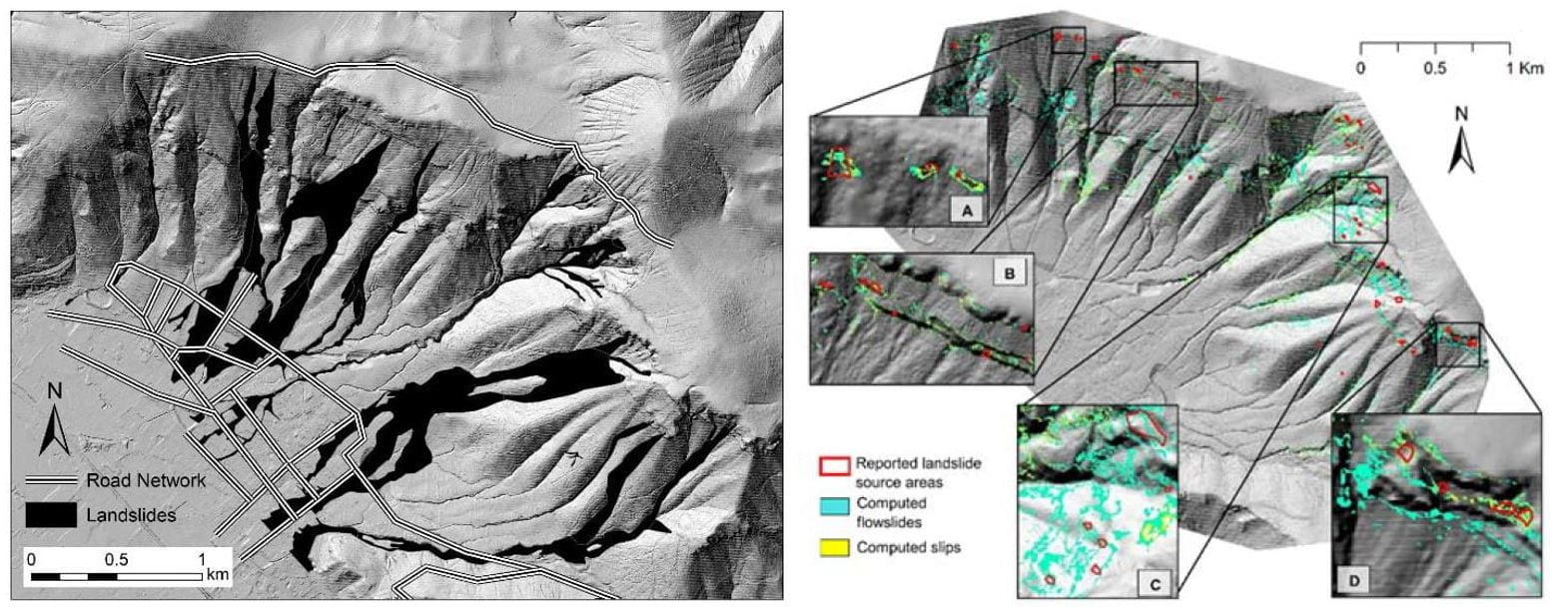
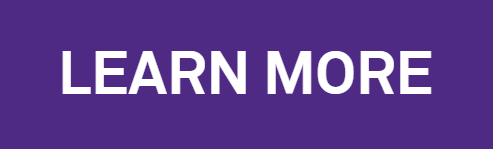
Simulation of long-term land subsidence
Unregulated use of water and energy resources is among the key causes of land subsidence, a phenomenon which in conjunction with sea-level rise can greatly exacerbate flood risks for coastal communities. Research done at the SPREE center develops data-driven computational platforms to simulate the long-term development of ground settlements in areas affected by anthropogenic subsidence.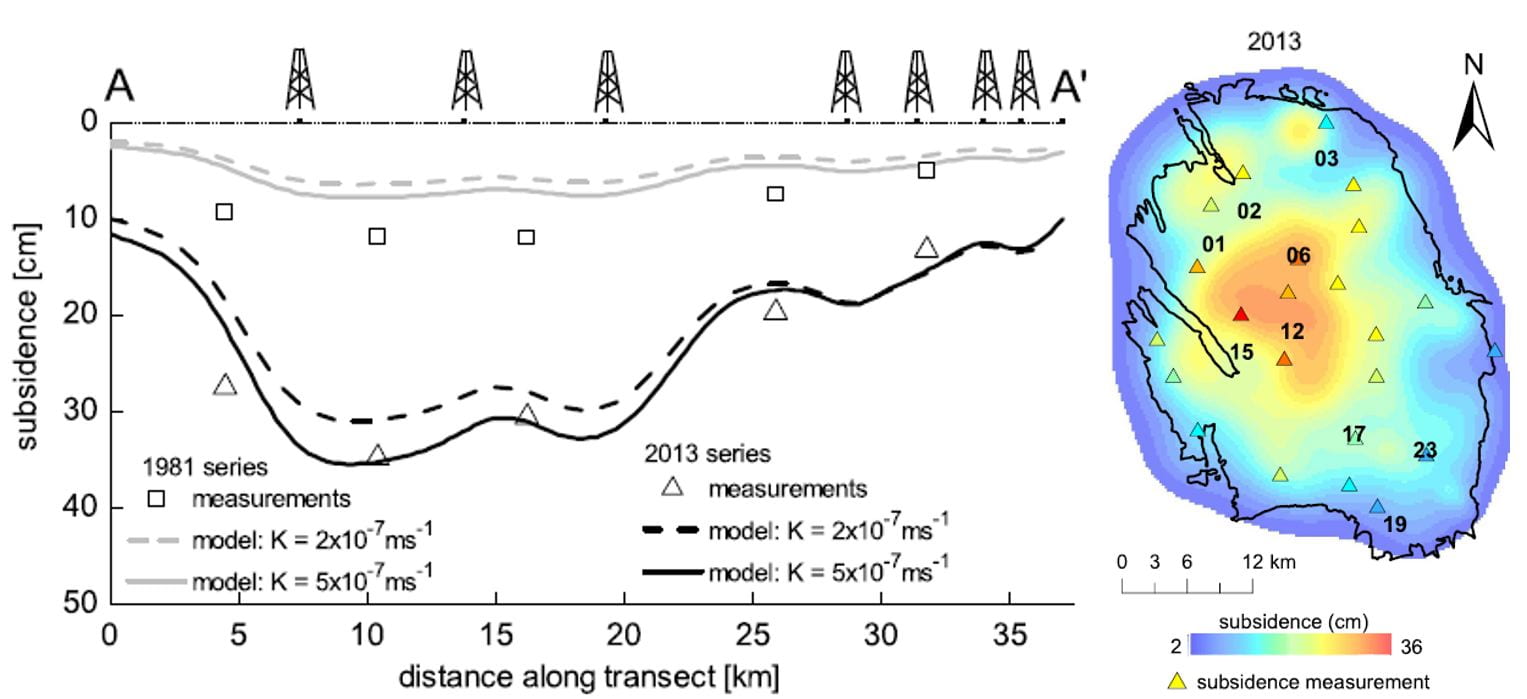
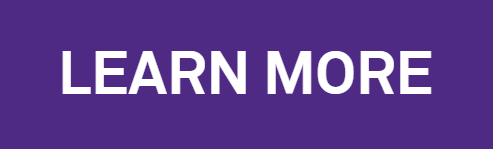
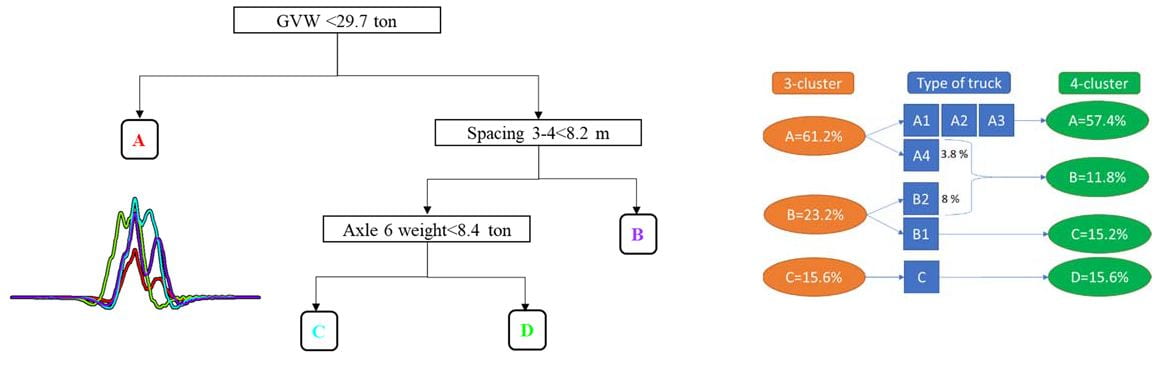
Infrastructure worldwide (and particularly in the United States) continues to age and require maintenance and/or replacement, at a time when infrastructure budgets are highly constrained. At the same time, computational power and sensor technology continues to advance. These two forces combine to create a unique situation where the application of sensing and computation to infrastructure management provides opportunities for efficiency and safety that have not previously existed. However, there are challenges to this framework that thus far limit the adoption of these technologies to (i) Data management and information distillation and (ii) Design of monitoring systems.
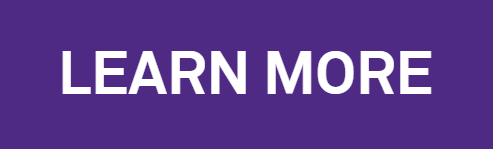
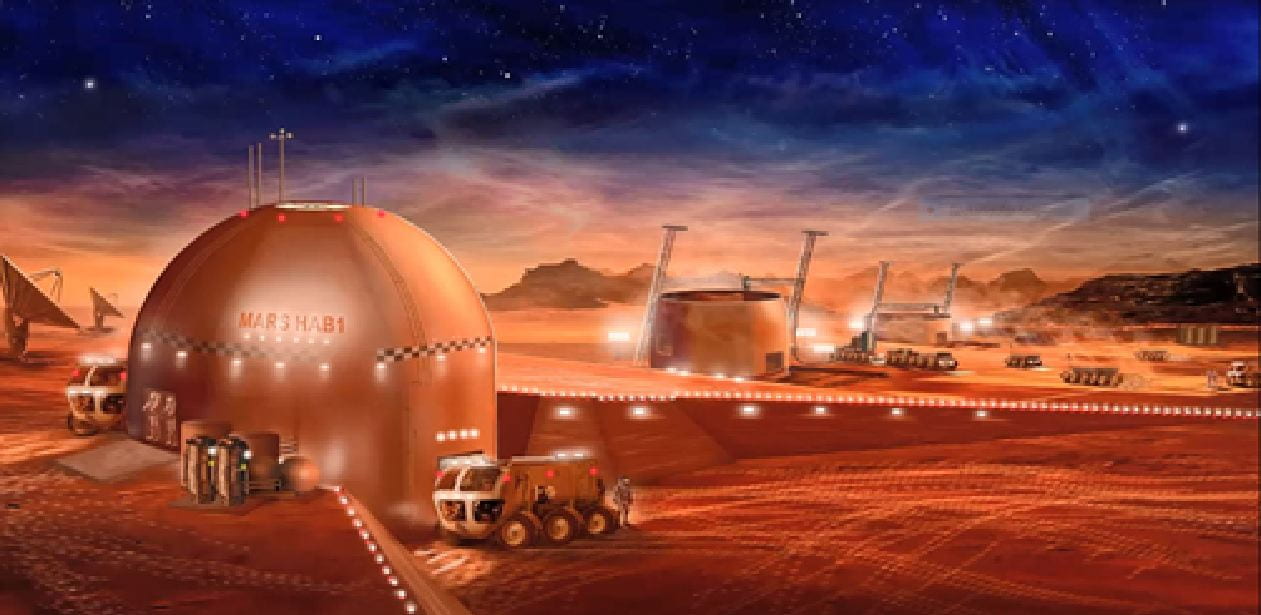
Artist’s rendering of future construction on Mars (image credit: NASA).
The SPREE Center’s thrust in construction automation explores potential advances in the analysis, design, construction, and use of infrastructure brought about by rapid growth in computing and robotics. In addition to enabling efficient new forms of construction—both on Earth and other planets—these advances will bring a number of impacts on the CEE workforce, both positive and negative. This thrust explores not only enabling technologies in construction automation but also the impacts of technological advances on the CEE workforce, devising strategies to best educate future civil and environmental engineers in an era of rapid change.
Whether or not computers can replace humans in the complex work of engineering design is a question that transcends CEE and has received considerable attention with advances in computing, particularly in the area of artificial intelligence. On the abstract level of mathematics and computer programming, the engineering design process can be viewed as the solution of extraordinarily complex problems in constrained optimization. For example, a quintessential endeavor in structural engineering typically involves selecting the configuration and materials that minimize cost subject to constraints imposed by safety, functionality, design life, constructability, and numerous other factors. At present, this design process is completed by structural engineers with training in basic mechanics as well as the application of design codes that have evolved over many years through various professional organizations. It is becoming less and less difficult to imagine that much of this intellectual work could be replaced by computer algorithms as designers rely increasingly on simulation-based engineering and virtual prototyping.
Similarly, the extent to which robots will perform physical tasks that are currently done by humans has become a pervasive area of interest and speculation. While increasingly sophisticated robots have been taking over routine physical work in factories for decades, recent advances in artificial intelligence have suddenly equipped machines with cognitive capabilities that enable them to accomplish activities once considered ‘mission impossible,’ such as reading and driving. Successes playing out in autonomous driving motivate and inspire numerous other applications of robotics in CEE. Could autonomous vehicles equipped with sensors be used to detect deficiencies in roads (i.e., infrastructure health monitoring)? In what ways will advances in robotics revolutionize the tools and processes used to build the road itself? Could earthworking be completed by distributed systems of machines much like ants build their colonies? How might robotics enable the construction of new, high-performance pavements (e.g., using functionally graded materials)? Robotics already provides a framework for the widespread adoption of additive manufacturing (AM), popularly known as 3D printing, with numerous possible extensions to CEE. AM allows for the design and production of complex shapes that liberate the imagination of designers, architects, and engineers, opening unexplored possibilities for performance optimization to achieve stronger, tougher, more durable, more aesthetically appealing, and more environmentally friendly products, while possibly even reducing costs. The adoption of AM in CEE is hampered by technological hurdles associated with extending the process to large scales and materials other than polymers (plastics).
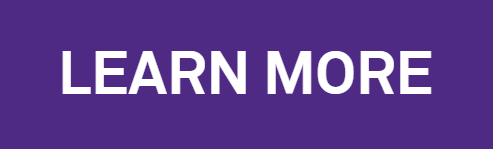
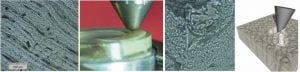
The vision of the Bioinspired Engineering thrust is to apply physical sciences to address pressing public health issues and pressing civil engineering needs via a multiscale interdisciplinary approach. To this end, we aim to (i) characterize and predict the behavior of biological systems, (ii) create biomimetic systems, and (iii) leverage the synergy of biological systems to enhance civil engineering infrastructure.
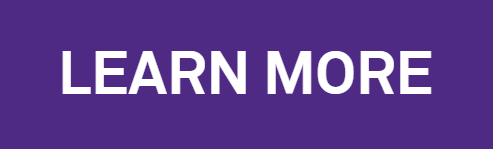
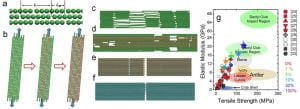
Load-bearing (structural) engineering materials are traditionally thought of as static, having little kinetic and multifunctional features. Conversely, many natural and biological systems blend structural capabilities with other uses such as fluid transport and stimuli-responsiveness, and exhibit kinetic architecture stemming from molecular and multi-scale hierarchical features. The objective of this thrust is to establish modeling, simulation and experimental analysis tools to design novel structural materials with unforeseen dynamic and multifunctional capabilities.
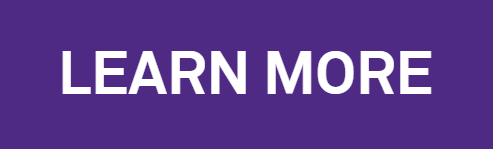
Granular Materials at High Pressure
Granular materials are ubiquitous elements of the natural world and are involved in disparate technological applications. At the SPREE center, we study the engineering properties of granular materials across length and time scales by developing methods to characterize and simulate their mechanical performance. An example is the deformation and failure of granular systems subjected to high confining pressure, such as those taking place during penetration, impact, and confined compression. We study such phenomena in relation with changes in the distribution of particle size and shape within assemblies exhibiting grain crushing, i.e. one of the most disruptive changes of the microstructure of this widespread class of engineering materials.
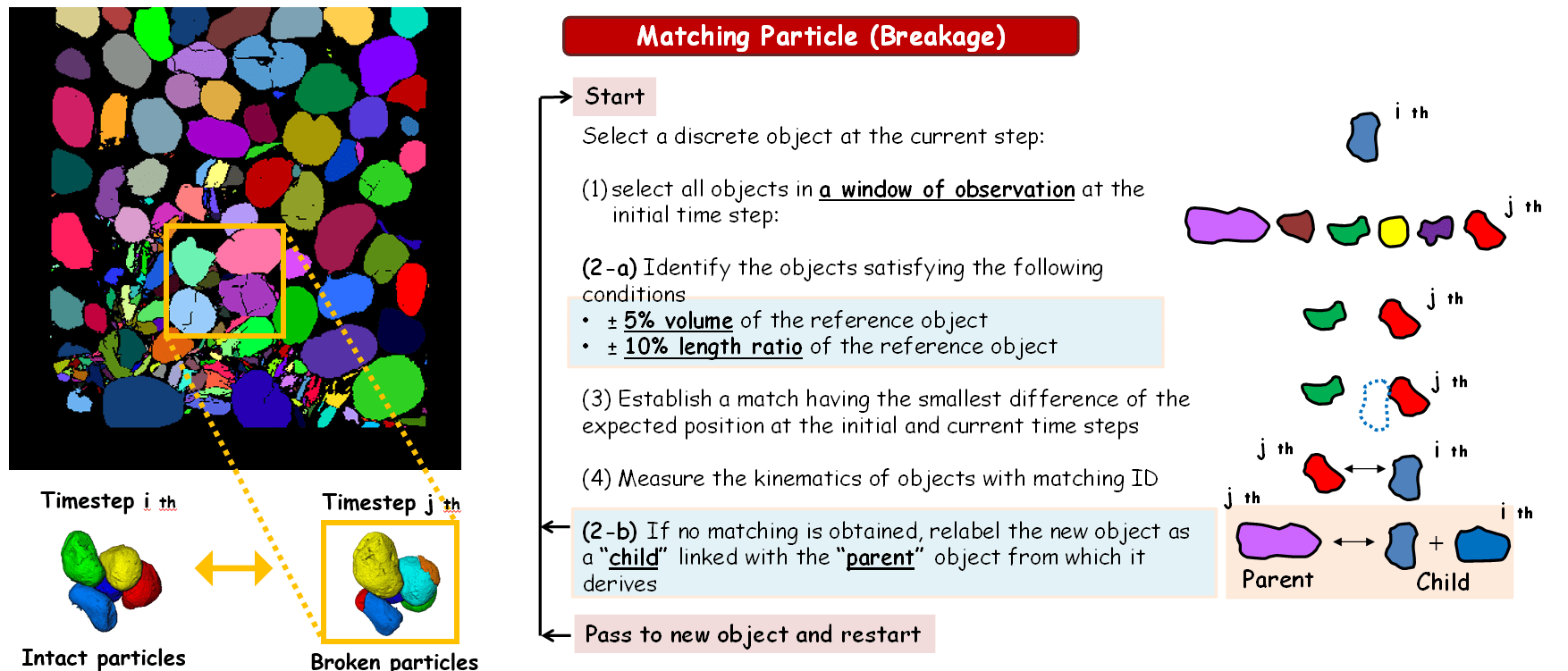
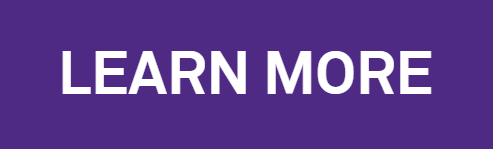
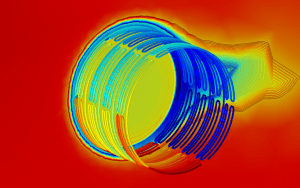
Energy is increasingly required to support human activity and development worldwide. In many instances, the harvested energy sources to meet such activity and development are non-renewable and are accompanied by poor management and reutilization strategies. This evidence involves detrimental consequences for natural and built environments, such as environmental pollution, extreme weather, and natural hazards, with alarming scenarios for the future. Engineering science can play a key role in the conceptual development, analysis and design of technologies, systems and methods that can allow for an effective harvesting, management and reutilization of renewable energy sources. This thrust focuses on the development of fundamental scientific knowledge to assist in the previous endeavor. Specifically, through the development of theoretical knowledge and experimental evidence, this thrust aims to transform current approaches to engineer, analyze and design materials and structures for the clean and efficient harvesting, storage and reutilization of energy resources. The ultimate goal of such activities is to deploy energy solutions that can sustainably meet human activity needs with a limited impact on the environment.
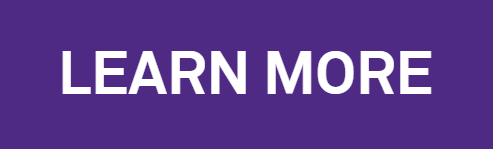
Heterogeneous Compaction of Porous Rocks
High-porosity rocks host the largest fraction of water and energy resources in the planet and are common targets for the deep geological storage of gas and liquid by-products. Given their susceptibility to faulting, being able to simulate their response to changes in stress state due to natural tectonics and anthropogenic injection/extraction of fluids is strategic for modern society. At the SPREE center, we study the mechanics of rock compaction across multiple length scales, ranging from the individual forming their solid skeleton to the outcrops affecting regional fluid conductivity. Specifically, we formulate mathematical indicators of impending localized compaction, as well as computational models replicating the spatiotemporal patterns of formation and propagation of heterogeneous compaction zones.
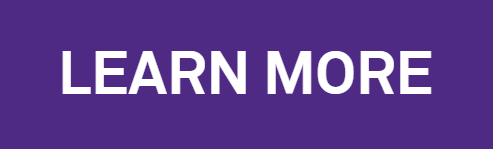